BHSI note: This is Dr. Voigt Hodgson's first study of bicycle helmets. We also have his more
definitive second study, done in 1991, with considerably more info on sliding resistance, more variables tested and
more extensive conclusions.
This study is also in .pdf format if that works better for you.
Impact, Skid And Retention Tests On A
Representative Group Of Bicycle Helmets
To Determine Their Head-Neck
Protective Characteristics
Voigt R. Hodgson, Ph.D.
Director Biomechanics Laboratory
Department of Neurosurgery
Wayne State University
Detroit, Michigan
Wayne State University
Department of Neurosurgery
550 E. Canfield
Detroit, Michigan 48202
February, 1990
This report was prepared in cooperation with the Michigan Bicycle Helmet Advisory Committee and the Michigan Department
of Public health.
The study was funded thru a grant, R49/CCR 503309-1, from the Centers for Disease Control, U.S. Public Health Service.
Additional support was provided by Wayne State University Neurotrauma Prevention Fund.
ACKNOWLEDGMENTS
Albert I. King was principal investigator for the Centers for Disease Control project and this
work was done at his suggestion and encouragement.
L. M. Thomas, M.D., as chairman of the Department of Neurosurgery, keenly supported this research.
Ken Thompson helped devise the instrumentation to make the measurements and performed the computer operations necessary
to produce this document.
Jamie Mayes laid out the illustrations and tables and performed the word processing.
Matthew Mason performed the high speed and still photography, and built the skid test setup.
Eugene Dupuis and Robert Neumann assisted in the instrumentation and mechanical setups associated with the tests.
Page i
ABSTRACT
A group of ten bicycle helmets, representative in size, style, and cost of those which would be suitable
to participate in a youth helmet use encouragement program, were evaluated for safety characteristics. This included
retention tests, drop impact tests onto flat and curved rigid surfaces, and skid impact-tests against a concrete slab
mounted at various angles. The helmets included both no-shell and hard shell types, six which met the ANSI Z90.4
Standard, which has a 39.4 inch (1 meter) drop requirement and four which met the Snell Standard, requiring 78.7 inch (2
meter) drops .
It was found that all retention systems were adequate in strength and stretch characteristics for uniform loading, but
that they were easily rotated on the head if loaded unsymmetrically.
A 39.4 in (1 m) drop with no protection onto any of four locations on the humanoid head model produced a range of from
70% to >99% risk of serious injury response in the model. Wearing any of the helmets reduced the risk of serious
injury to 1% or less for 39.4 in (1 m) drops onto either surface and almost all locations.
For 78.7 inch (2m) drops there were significant risks of serious head injury for most conditions, although two helmets
showed that on average the risk could be held to 6%.
The skid tests, utilizing a head-neck instrumented Hybrid III Dummy, concrete slab tilted at several degrees to the dummy
head path, high speed cinephotography, and conducted at 6 mph (9.7 km/hr), revealed that there was a significant
difference in effect on the head and neck injury potential between the hard and no-shell helmets for 30 and 45 degree
angles, but at 60 and 90 degrees there was no significant difference. Both types of helmets produced unique head-neck
injury hazards. A clear plastic face shield would help reduce the injury risk of both. Further study is needed to
determine more comprehensively which type helmet is most safe, but in the meantime use of either should be encouraged as
being much safer than bicycle riding without a helmet.
Page ii
TABLE OF CONTENTS
ACKNOWLEDGMENTS........i
ABSTRACT........ii
I. INTRODUCTION........1
II. TEST PROCEDURES........2
2.1 Drop Tests........2
2.2 Skid Tests........2
Figure 1: Drop Test Setup........3
Figure 2: Head Injury Parameters for Direct Head Impact........4
Figure 3: Skid Test Setup........5
2.3 Retention Tests........6
III. RESULTS
3.1 Drop Tests........6
Figure 4a: Static Helmet Retention System Strength Test Setup........7
Figure 4b: A Dynamic Retention System Strength Test........7
Table 1: Bicycle Helmets in Head-Neck Protection Test Program........8
Table 2: Bicycle Helmet Drop Test Results-Small Size 39.1 in (1m)........9
3.2 Skid Tests........10
Table 3: Bicycle Helmet Drop Test Results-Small Size 59.1 in(1.5m)........11
Table 4: Bicycle Helmet Drop Test Results-Small Size 78.7 in(2m)........12
Figure 5: Skid Test of a Bare Headed Hybrid III Dummy........13
Figure 6: Skid Test of a No-shell Helmet........14
Figure 7: Skid Test of a Hard shell Helmet........15
Figure 8: Skid Test of a Hard shell Helmet With Face Shield........17
Figure 9: Dummy Head-Neck Skid Test Response in Typical Hard Shell Helmet........18
Figure 10: Dummy Head-Neck Skid Test Response in Typical No-Shell Helmet........19
Table 5: Typical Bicycle Helmet Skid Test Results at Several Angles........20
Table 6: Bicycle Helmet Skid Test Results 45 Degree Angle to Concrete........21
3.3 Retention Tests........22
IV CONCLUSIONS........22
REFERENCES........25
Page iii
Impact, Skid And Retention Tests On A
Representative Group Of Bicycle Helmets
To Determine Their Head-Neck
Protective Characteristics
Voigt R. Hodgson, Ph.D.
I. Introduction
Head injuries serious enough to cause death or permanent memory, intellectual and motor
impairment among children riding bicycles, is a growing health problem (Reference 1). According to Dr. Mark Widome,
Chairman of the American Academy of Pediatrics Committee on Accident and Poison Prevention, bicycle related head trauma
accounts for 80 percent of 500 children who die each year riding bicycles, and an additional 70,000 are treated in
emergency rooms each year for bicycle related head trauma (Reference 2). Widome estimates that helmets would prevent
three out of four serious head injuries to children. A field study by Thompson, et al. (Reference 3), comparing helmeted
and unhelmeted rider bicycle accidents, estimated that wearing bicycle helmets decreased the risk of head injury by
approximately 78%. However it is estimated that less than two percent of children between the ages of 5 and 14 years wear
protective helmets (Reference 4).
This head and neck injury research program was instituted to provide evidence from an experimental perspective, which
quantifies the risks of injury with and without a helmet. Hopefully this information will be useful in campaigns to
encourage helmet use among children and thereby be influential in campaigns to encourage their use among young bicycle
riders. Among the helmets selected for this study were some which have met the Snell Memorial Standard (Reference 5),
having a 78.7 inch (2 meter(m)) drop requirement, as well as those which have met the ANSI Z90.4 Standard (Reference 6)
(39.4 inch (1m) drops). In each of these categories were chosen several of the traditional hard shell and more recently
introduced no-shell helmets. All helmets were tested for their ability to 'protect' a humanoid headform for impacts
perpendicular to rigid flat and hemispherical shaped surfaces from drop heights of 39.4 inches (1m) to 78.7 inches
(2m).
The child size humanoid headform made it possible for the first time to: a)compare and make available the response of the
bare head to that of the helmeted headform, for drops from a height typical of a child's fall from a bike; and, b) use a
head injury criterion level (References 7 and 8) vs. serious head injury risk probability curve (Reference 9) to compare
the protective performance of helmets against human tolerance. Some tests with a modified full sized dummy, instrumented
with head-neck transducers, were later added to the program to evaluate the potential for risk of head and neck injury
due to the so called 'hangup' effect
Page 1
caused when the helmeted or unhelmeted head strikes the pavement with significant forward velocity.
II. Test Procedures
Four Series of tests were conducted, including:
2.1 Drop Tests - A small size 6 5/8, humanoid head form instrumented with a triaxial accelerometer for measuring
resultant linear head center of gravity accelerations from which Severity Index (SI) [Footnote a below this paragraph]
was computed, were dropped onto rigid surfaces to simulate a sudden unimpeded impact of a child's head with the pavement
(Figure 1). These tests were conducted from 39.4, 59.1, and 78.7 inches (1, 1.5, and 2m), onto rigid flat and hemispheric
shaped 2 inch (50mm) radius surfaces with impact on front, side, rear and top surfaces. The helmets all had as their
primary energy absorbing material, expanded polystyrene (eps). Each impact was against a part of the helmet which had not
previously been impacted.
where T is effective impact duration.
A is instantaneous acceleration (G).
dt is time increment of integration.
Risk of injury was evaluated according to the Severity Index and use of the risk chart (50% line) shown in Figure 2
(Reference 9). This curve was constructed from the only available evidence worldwide, linking the percentage of adult
population likely to be at risk of a serious head injury for a given level of linear head acceleration impact response.
The data were derived from head impacts received by adult cadavers in controlled falls attempting to produce linear skull
fracture in some cases and simulated arterial hemorrhage in others, while measuring linear head accelerations on the
skull. Since the risk of both of these end points from a head impact increases with age, the risk derived from this curve
is probably conservative for most youths. However because of the uncertainty of individual human tolerances and the fact
that rotational motion superimposed on the linear motion of the head is likely to lower the impact tolerance of an
individual, it is best to be on the conservative side when estimating the protective performance of a helmet.
2.2 Skid Tests - A group of no-shell and hard shell medium-sized helmets were
Page 2
FIGURE 1
Drop Test Setup Showing Small Humanoid Head Model With No Shell Helmet in Position for Side Impact to
Rigid Flat Anvil. The 2 inch(50 mm) Radius Rigid emisphere Impact Surface is Also Shown.
Page 3
Page 4
FIGURE 3
Skid Test Setup With No-Shell Helmet Mounted on the Hybrid III Dummy in Position to Contact a Concrete
Slab Attached to a Force Transducer and Inclined at 45 Degrees to the Horizontally Driven Dummy.
Page 5
mounted on a Hybrid III dummy which was reduced in weight to 130 lb (59Kg) by removal of arms and legs (Figure 3). The
dummy was driven horizontally by spring propulsion to free wheel at 6 mph (9.7 km/hr) into a concrete barrier inclined at
angles of 30, 45, 60, and 90 degrees to the horizontal. The dummy torso was at an angle of attack of 15 degrees to the
horizontal and the neck of the dummy was in horizontal alignment. The helmets struck the surface at an initial contact
point 3.9 inches (100 mm) above the front rim edge for the 45 degree tests and at a contact point determined by the
barrier tilt for impacts below and above 45 degrees. Only this dummy orientation was examined. The dummy was instrumented
with a linear triaxial and an angular accelerometer in the head and a six channel, 3-axis force-moment transducer at the
head-neck junction. Video filming of selected skid tests were conducted to determine if there was visually observable in
real time evidence to support reports of no-shell helmets causing head-neck injury to riders in falls due to 'hang-up' on
the pavement (Reference 10); and, whether there is a difference in hang-up between hard and no-shell helmets. In
addition, high speed filming at 500 fps was conducted on selected tests to further clarify the issue of hang-up.
2.3. Retention Tests - Two types of tests were conducted: a) A quasi-static test with uniform loading around the bottom
rim of the helmets to test strength and deformation, ie, a 300 lb (136Kg) upward load on the rim must be held by the
retention system for 1 minute and not stretch greater than 1 inch (25 mm) (Figure 4a); and, b) A dynamically imposed load
of 250-300 lb (114-136Kg) applied to the retention strap under the chin must not cause either mechanical failure or
stretch in the retention system of more than 1 inch (25 mm) (Figure 4b).
III. Results
3.1 Drop Tests - Table 1 shows the distribution of youth bicycle helmets used in these tests in
terms of cost, standard met, type of outer surface, weight, center of gravity location, and mass moment of inertia about
a lateral axis. The Snell Standard test is assumed to identify safer helmets than the ANSI Z90.4 Standard, because it
requires a similar performance for 78.7 inch (2m) as compared to 39.4 inch(1m) drops.
Given in Table 2 are the 39.4 inch (1m) drop test results. Of particular significance to note in this set of data is the
comparison given between the head model response without and with any of the ten helmets. According to the best estimate
line in Figure 2 corresponding to the recorded Severity Indices, there would be a risk of serious head injury to 70
percent of the population for side impact, and to greater than 99 percent for the other three locations. For eight of the
10 helmets, the Severity Index computed from the model impact response predicts between less than 1 and 1 percent of the
population would be at risk for impacts on any location to either flat or hemi rigid surfaces.
Page 6
Figure 4
A. (top) Static He/met Retention System Strength Test Setup, in Which the System is Required to
Withstand a 300 lb (1.33 kN) Pull for 1 Minute Without Failure or Stretching More Than 1 inch (25 mm).
B. (bottom) A Dynamic Strength Test Was Also Performed in Which the 14 lb. (6.4 kg) Helmeted Head-Carriage Assembly was
Dropped Upside Down From a Height of 36 Inches (0.9 m) (42 ft-lb-57 J) and Arrested Through the Retention Strap Under the
Chin. In This Test the Tensile Force (Head Mass x Max. Deceleration - G) Varied From 224-308 lb (1 kN - 1.37 kN) With No
Greater Than 1 inch (25 mm) Stretch.
Page7
Page 8
Page 9
Table 3 contains the 59.1 inch (1.5m) drop data. Even at this intensity, risk levels are relatively low, not exceeding 5
percent except in the two worst cases, which rose to 12 percent.
Table 4 contains the 78.7 inch (2m) drop test results and at this level all of the helmets produced a significant risk
level at some location. Averages and standard deviations for each helmet are given in the two far right columns, whereas
the statistics across the array of helmets are given below each column. Helmet 'A', made by a manufacturer known to be
interested in participating in helmet encouragement programs, had the lowest combined average risk and standard deviation
among the helmets.
Although the Snell helmets (A, B, C, G ) generally produced lower risk scores than those which meet the ANSI Z90.4
Standard, helmet F, which meets the ANSI Standard, was a close second lowest risk to helmet 'A'.
3.2 Skid Tests - Figures 5-8, which are excerpts taken from 16 mm film recorded at 500 fps, illustrate dramatically the
effect of the two types of protective helmets on the head and neck for impact at 6 mph (9.7 km/hr) to a 45 degree
concrete angle. In Figure 5, with no helmet, the rubber covered dummy head does not slide up the ramp but rather rolls
like a wheel, putting extreme flexural and axial stress on the neck. This can be visualized by observing the increase in
neck curvature from frame one at initial touch to maximum flexion before rebound in frame three. This neck, although not
similar in structure to the human neck, has been designed to simulate the response of a human neck held in rigid
anticipation of an impact to the body which produces bending in either flexion or extension (11). Although the dummy head
is soft rubber coated, the human head would tend to grip the pavement in a similar manner depending on surface
roughness.
In Figure 6 are shown a similar set of frame sequences for a skid test with the dummy wearing a typical no-shell helmet.
The motion is similar to the no helmet case shown in Figure 5, in that no skid occurs. Instead the head rolls up the
ramp, loading the neck in flexion and axial compression, predicting neck injury as the primary hazard, since head
accelerations were minimal.
Figure 7 illustrates the effect of a typical hard shell helmet on the dummy head impact to the 45 degree concrete ramp at
6 mph (9.7 km/hr). There is initial gripping of the concrete by the helmet shell, causing momentary neck flexion, after
which it breaks loose, allowing the head to clear the surface as the neck extends, and the motion culminates with the
face slamming down on the concrete and hanging up to produce a second period of neck flexion. The facial
Page 10
Page 11
Page 12
FIGURE 5
A Sequence of Enlarged frames Taken From 16 mm Film at 500 fps Showing the Skid Test of the Bare Headed
Hybrid /// Dummy Hitting the Concrete Ramp at 45 Degree, 5 mph ( 8 km/hr).* The Friction Force Between Head and Concrete
Was Too Great to Allow Skid, Causing The Head to Roll, Flexing and Axially Loading the Neck, High Angular Acceleration
and Rebound From the Slab.
*Nominal value - later confirmed to be 6 mph (9. 7 km/hr) as given in Tables 5 and 6.
Page 13
FIGURE 6
A Sequence of Enlarged frames taken From 16 mm film at 500 fps Showing the Skid Test of the No Shell
Helmeted Head of the Hybrid III Dummy Hitting the Concrete Ramp at 45 Degrees, 5 mph (8 km/hr).* The Friction Force
Between Helmet and Concrete Was Too Great to Allow Skid, Causing The Head to Roll, Flexing and Axially Loading Neck,
Moderate Angular Acceleration and Rebound from the Slab Similar to the No Helmet Case Shown in Figure S
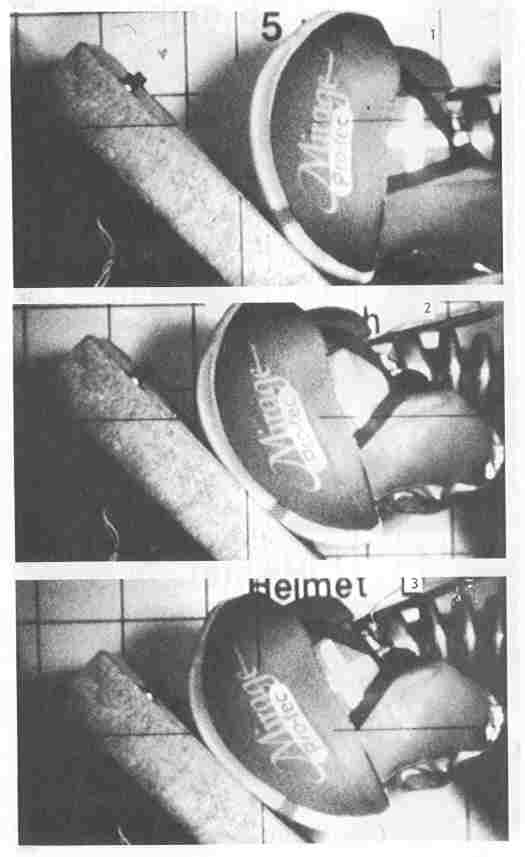
*Nominal value - later confirmed to be 6 mph (9.7
km/hr) as given in Tables 5 and 6.
Page 14
FIGURE 7
A Sequence of Enlarged Frames Taken From 16 mm Film at 5QO fps Showing the Skid Test of a Hard Helmet on
the Hybrid III Dummy Hitting the Concrete Ramp at 45 Degrees, 5 mph (8 km/hr) *: The Friction Force Between Shell and
Surface Momentarily Gripped Causing Flexion and Axial Loading of the Neck; The Helmet Then Broke Loose, Clearing Surface
and the Head Finally Slammed Down on the Face Causing High Angular Acceleration of the Head.
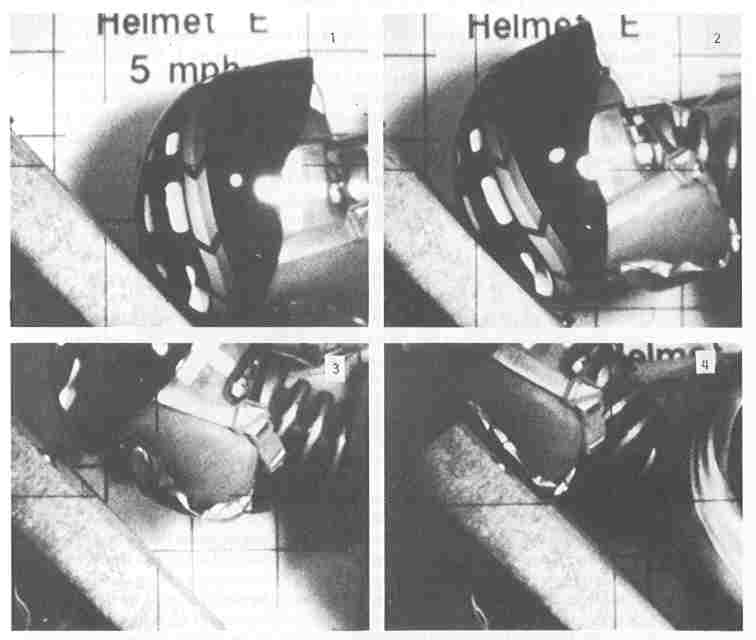
*Nominal value - later confirmed to be 6 mph (9.7 km/hr) as
given in Tables 5 and 6.
Page 15
friction causes high angular head acceleration to occur, at a level shown on the right side of Figure 2 to be near that
estimated to have the potential to rupture a bridging vein between brain and skull (Reference 12). Consequently, for the
hard shell helmet sliding on concrete under similar conditions, brain hemorrhagic and/or severe facial injury would be
the primary hazards.
Figure 8 illustrates a safety measure which could be applied to the hard shell (and possibly to the no-shell) helmet
situation shown in Figure 7. The addition of a hockey face shield to a typical hard shell helmet not only saved the face
of the dummy sliding in contact with the concrete, but the low friction of the shield eliminates the high angular
acceleration which would otherwise occur when the face hits the concrete. Furthermore, the chin pad bearing face shield,
in addition to impact protection, may be more comfortable than an under the chin strap with buckles. It may also provide
better stability for retaining the helmet in place during riding and impact than the retention systems which were all
rather similar among the representative group of helmets in this test program.
Figures 9 and 10 illustrate the differences in head-neck response for the hard shell and no-shell helmets, respectively,
for the 45 degree, 6 mph (9.7 km/hr) impacts. Of particular significance is the much longer (150-175ms) flexural bending
moment and axial neck loading for the soft shell (Figure 10), compared to only approximately 30 ms for the hard shell
impacts. However the hard shell helmet is not without its risk of injury causation. The relatively larger angular
acceleration (third from bottom trace) experienced initially by the dummy wearing the hard shell helmet (compared to the
no-shell helmet), was due to momentary hang-up of the hard shell on the pavement, followed by sudden release as the
friction force was overcome by body inertia. Secondary head angular acceleration occurred when the face slammed down on
the slab.
It must be emphasized that the risks predicted for head and neck injury in a skidding type fall while wearing either hard
shell or no-shell helmet were found to be much worse in every respect when no helmet was worn on the dummy. (See Table 5
and Figure 5.) Also, the differences in response and injury risk between the two types of helmets appears to occur over a
narrow range of angular approach to the concrete, being observed primarily between 30 and 45 degrees for this impact
speed of 6 mph (9.7 km/hr). Table 5 shows that for ramp angles of 60 and 90 degrees, the type of helmet worn makes no
significant difference when comparing results obtained from representative no-shell and hard shell helmets, at 6 mph (9.7
km/hr). For 30 degree, 6 mph (9.7 km/hr) impacts, the head and neck injury criteria are all well below injury level for
either type of helmet, but no-shell helmets produced higher neck loads and head accelerations due to hang-up. Additional
tests are planned to compare the relative hazard to
Page 16
FIGURE 8
A Sequence of Enlarged Frames Taken from 16 mm Film at 500 fps Showing the Skid Test of a Hard Shell
Helmet With Clear Poly Carbonate Hockey Type Chin Bearing Face Shield Hitting the Concrete Ramp at 45 Degrees, 5 mph (8
kmlhr). The Film Shows How the Motion Is Similar to that of Figure 7 (without the face shield) But the Face Shield Not
Only Saves the Face From Laceration and Possible Fractures But Eliminates High Angular Head Accelerations Which Would
Otherwise be Caused by Friction Forces When the Face Slams Down on the Concrete.
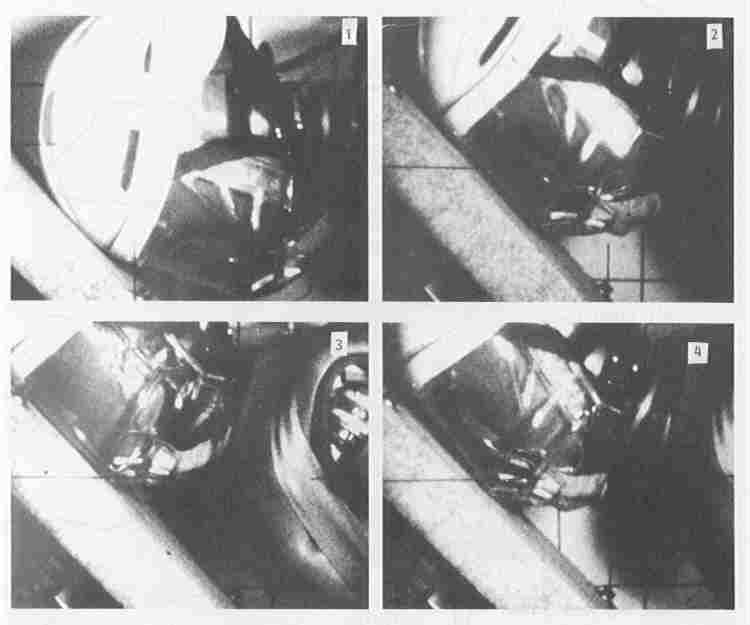
*Nominal value - later confirmed to be 6 mph (9.7 km/hr) as given in
Tables 5 and 6.
Page 17
FIGURE 9
Helmet J Typical Hard Shell Helmet Response of Head-Neck Transducers In Concrete Barrier 45 Degree, 6
mph(9.7 km/hr) Impact
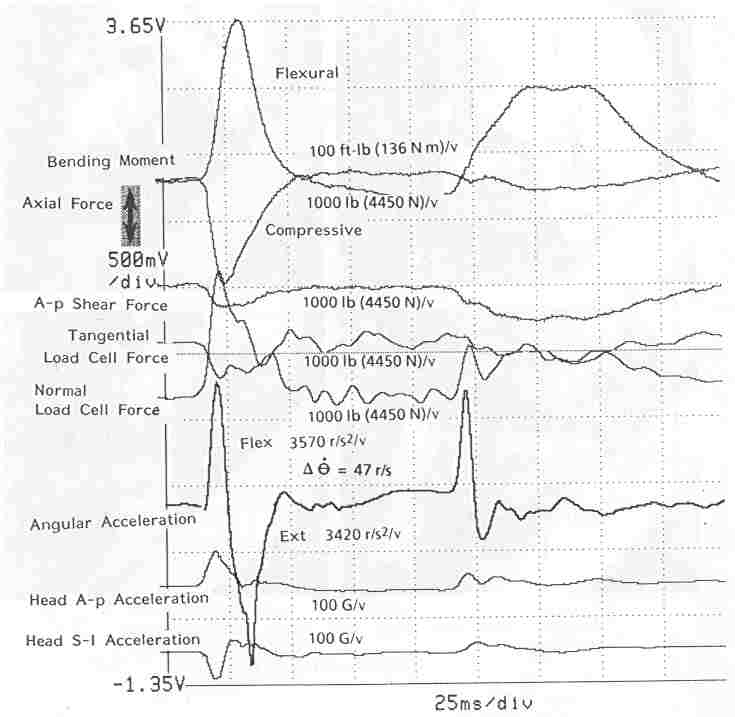
Interpretation:
Hard shell helmet grips concrete for approximately 35 ms, causing neck flexion, high head angular acceleration and low
linear acceleration. The hybrid III dummy body inertia forces the helmet loose and the neck extends, causing high angular
acceleration reversal and the head to bounce clear off the flexion accompanied by angular acceleration for 10-15 ms.
Total secondary flexion is 100 ms.
Page 18
FIGURE 10
Helmet J Typical Soft Shell Helmet Response of Head-Neck Transducers In Concrete Barrier 45 Degree, 6
mph(9.7 km/hr) Impact
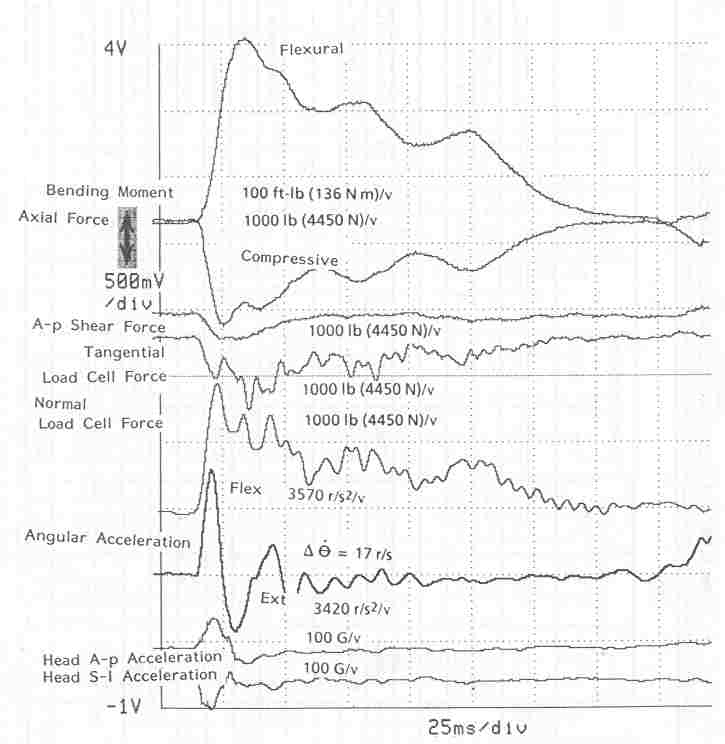
Interpretation:
The no-shell helmet grips the concrete causing high initial neck flexion and axial load, peaking at approximately 20 ms
and gradually declining together to zero at approximately 175 ms at which time dummy rebounds. Angular acceleration is
high initially with low reversal neither near critical, together with very low linear head acceleration.
Page 19
Page 20
Page 21
head and neck of the two types of helmets in low trajectory angle impacts with the simulated pavement at higher
velocities necessary to reach injury level.
The results shown in Table 6 verify that both the no-shell and hard shell helmets perform rather consistently like their
counterparts when comparing critical head (angular acceleration) and neck (bending moment and axial force) catastrophic
injury measurements. Also, the average amplitudes of these measurements for the two groups reflect the previous
discussion as to comparative injury hazard for the two types of helmets.
3.3 Retention Tests - All of the helmets exhibited adequate strength and stiffness for symmetrical force application, but
all tend to easily roll on the head when forced by non-symmetrical impact, especially if worn with loose straps. A chin
pad bearing face shield, strapped securely to the dummy, remedied this situation in the laboratory.
IV. Conclusions
Retention
1. All helmets met requirements for static and dynamic retention strength for uniform loading.
2. For non-uniform loading and loose retention, all helmets are easily displaced.
3. From a biomechanical standpoint, a face shield would serve the purpose of minimizing facial injury in sliding
accidents, provide retention stability with a chin cup, and reduce angular acceleration and neck bending caused by
friction between the face and impact surface.
Shock
4. With no helmet a 39.4 inch(1m) unimpeded fall to a rigid surface would be predicted by these results to cause a
serious head injury to 70-99% of the population. [Footnote 1 below]
5. Results predict that, wearing any of the helmets in this group, less than 1% of the population would be at risk of
serious head injury for such an accident.
Footnote 1. Based upon estimates obtained from surrogates representative of the general population of adults
(9).
Page 22
6. Results indicate that it is possible for a helmet to protect at relatively low risk levels [11 % or less (A)] for
78.7inch(2m) drops onto flat or 2 inch (50mm) radius rigid surfaces.
7. Degree of severity of the Standard met by a helmet was not a reliable indicator of safest performance. Among the
four lowest average risk helmets, in the 78.7 inch (2m) test, three (A, B, C) met Snell (78.7 inch-2 m drop ht. req.)
and one (F) met ANSI Z90.4 (39.4 inch-1 m). However the two helmets (H, l) scoring significantly higher than the
others, were both ANSI Z90.4 approved.
8. For 78.7 inch(2m) drops, there were very significant differences in protection afforded by this group of helmets,
varying from 6% - 35% best estimate the risk of serious head injury for the average of four impact locations.
Skid
9. Helmets with or without shells allow the head to slide after an initial hang-up period, for low angle trajectory
impacts into pavement (30 deg or less) at 6 mph (9.7 km/hr). Head injury indices and neck loads measured in the dummy
were below injury level. However the neck flexion bending, axial load and head angular acceleration, were generally
highest for no-shell helmets. Facial injury is predicted to be a hazard in either type helmet for this condition.
10. Wearing no helmet produced estimated injury level head angular acceleration and neck load measurements in the
dummy, for 6 mph (9.7 km/hr), 45 degree skids.
11. For the 45 degree concrete ramp impacts, no-shell helmets cause hang-ups in compression-flexion for longer periods
(175 ms compared to 30 ms), than do hard shell helmets, predicting more risk of neck injury in the no-shell
helmets.
12. Helmets with hard shells allow the head to slide out from neck entrapment in compression-flexion for trajectories
into pavement impact of 45 degrees at 6 mph (9.7 km/hr). However, they cause higher angular head accelerations and more
risk of facial injury for this condition than do no-shell helmets.
13. The 60 degree concrete ramp impacts at 6 mph (9.7 km/hr) produced excessive compression-flexion neck loads in the
dummy wearing either type of helmet.
14. Some no-shell helmets skid better than others because of more slippery covers or hard plastic retention strap
covers
Page 23
15. Significant differences in head-neck loading in the dummy, wearing no-shell or hard shell helmets for 6 mph (9.7
km/hr) front impacts, showed up only at ramp angles of 30 degrees and 45 degrees (most significant).
16. Effects of tangential loading on head and neck, with the dummy wearing no-shell vs hard shell helmets, requires
more thorough investigation in terms of surface friction, velocity angles, and impact location on the helmet to
determine which type helmet provides the best impact protection and what could be done to improve protection.
17. For 90 degree concrete barrier impacts on the crown at 4 mph ( 6.4 km/hr), there is essentially no risk of head
injury with either type of helmet or no helmet. However, excessive axial forces are produced with either type, with
only an 18 % reduction compared to no helmet.
18. Laboratory results show that wearing either type of no-shell or hard shell helmet typified by this group of
helmets, is much safer than riding a bicycle without a helmet and that either type would be safer and more stable
retained on the head by a chin bearing clear polycarbonate face shield.
Page 24
References
1. SAFE KIDS Bicycle Injury Fact Sheet, 1/17/89, Washington, D.C. 20010-2970
2. U.S. Senate Holds Landmark Hearing On Childhood Injury, Leadership Quarterly, Summer 1989, vol 1, Number 1, A
Publication of the National SAFE KIDS Campaign,111 Michigan Avenue, N.W. Washington, D.C. 20010-2970.
3. R.S. Thompson, F.P. Rivara, D.C. Thompson, Prevention of Head injury by Bicycle Helmets: A Field Study of Efficacy,
AJDC-Vol 142, April 1988.
4. Incentive Grants for Injury Control Intervention Projects, Michigan Pilot Project to Reduce Head Injury Among Children
involved in Bicycle Crashes, Center for Health Promotion Division of Research and Development, Health Surveillance
Section MAP, December 14,1988.
5. " 1984 Standard for Protective Headgear for Use in Bicycling", Snell Memorial Foundation, P.O. Box 733, Wakefield, RI
02880, 1984.
6. "American National Standard for Protective Head Gear - for Bicycles, American National Standards Institute, Inc.,1430
Broadway, New York, NY 10018, ANSI Z90.4, 1984.
7. Gadd, C.W.: "Use of a Weighted - impulse Criterion for Estimating Injury Hazard," Proceedings of the Tenth Stapp Car
Crash Conference, Society of Automotive Engineers, Two Pennsylvania Plaza, New York, NY, 1966.
8. Gadd, C.W.: "Report to SAE Performance Criteria Subcommittee," Vehicle Research Department, General Motors Research
Laboratories, G.M. Technical Center, Warren, Ml 48090, March 8,1972.
9. Prasad, P. and Mertz, H.J.: "The Position of the United States Delegation to the ISO Working Group 6 on the Use of HIC
in the Automotive Environment" Prepared for The Society of Automotive Engineers Technical Paper Series #851246, May
20-23,1985.
10. Are Foam-Only Helmets Safe? Bicycling Magazine, February 1989.
11. Mertz, H.J.; Neathery, R.F. and Culver, C.C.: "Performance Requirements and Characteristics of Mechanical Necks."
Human Impact Response, edited by William F. King and Harold J. Mertz, Published by: Plenum Press, New York, NY 1973.
12. Lowenhielm, P.: " Dynamic Properties of the Parasagittal Bridging Veins," Z. Rechtmedizin 1974, 74: 55-62.
13. "Human Tolerance to Impact Conditions as Related to Motor Vehicle Design - SAE J885, June 1985, a Report of the Human
Injury Criteria Task Force of the Human Biomechanics and Simulation Subcommittee (Revised June,1985), Published by:
Society of Automotive Engineers, Inc., 400 Commonwealth Drive, Warrendale, PA 15096.
14. Mertz, H.J.; Hodgson, V.R.; Thomas, L.M. and Nyquist, G.W.: "An Assessment of Compressive Neck Loads Under
Injury-Producing Conditions." Physician and Sportsmedicine, November 1978.
15. "Standard Method of Impact Test and Performance Requirements for Football Helmets," prepared by: National Operating
Committee on Standards for Athletic Equipment (NOCSAE), September 1973.
Page 25
(end of study)
Use this link for Dr. Hodgson's
more definitive second study, done in 1991.